The Bioelectric Network: A New Frontier in Understanding Intelligence and Morphogenesis
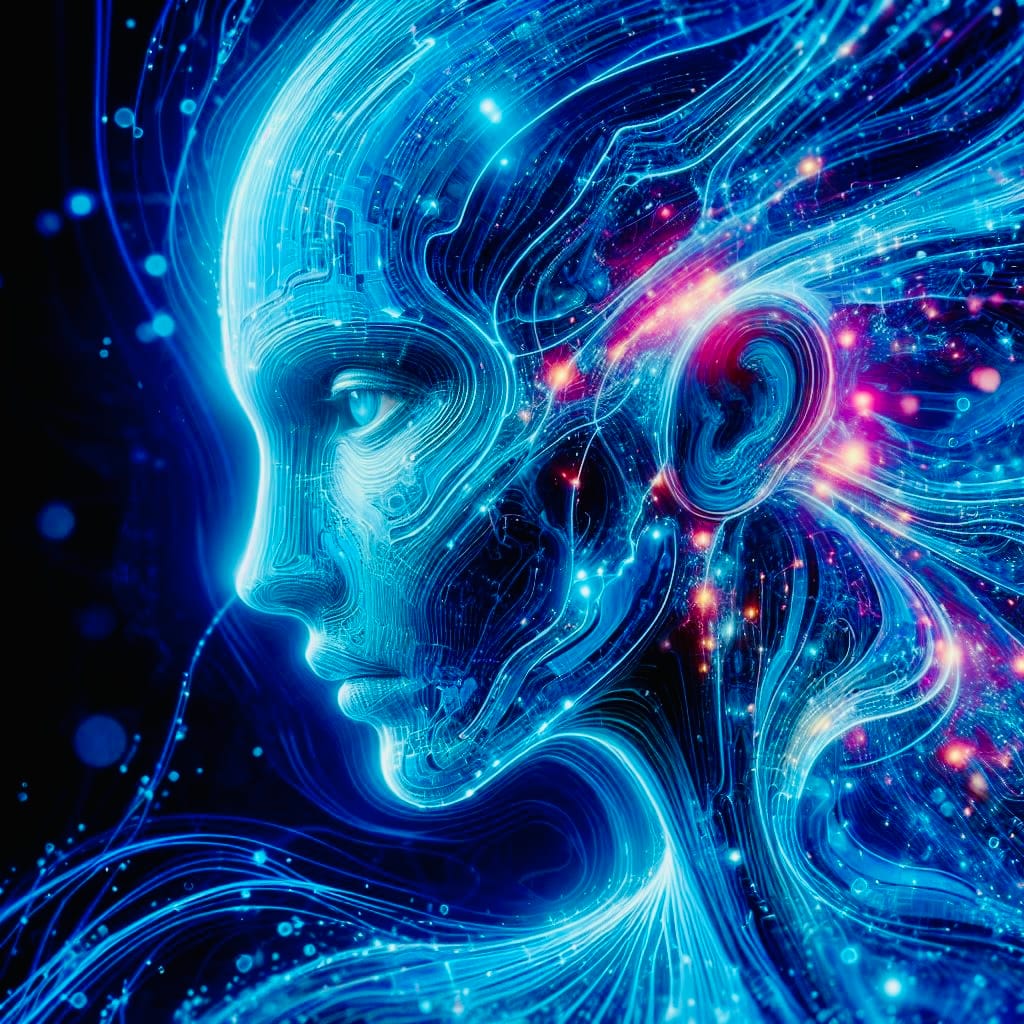
Where does the line get drawn between life and machines? What is the true root of animal intelligence? Is it possible to become immortal? These are questions that are gradually being demystified thanks to the research of Dr. Michael Levin. In this article, I will go over his research, explaining various concepts and the evidence behind them, as well as what it could mean for us as a species in the future.
Background: Understanding of the Primo Vascular System
Luigi Galvani, an Italian Physician and Physicist, made a groundbreaking discovery in the field of Bioelectricity in the late 18th century. He found that the muscles of dead frogs’ legs twitched as if alive when struck by a spark of electricity. This discovery led to the cementation of the concept “animal electricity”, or “Bioelectricity,” as it indicated that electrical forces existed within living organisms and are fundamental to life processes. Galvani’s discovery marked a pivotal moment in biology, blurring the line between physics and biology. This finding laid the foundation for the development of electrophysiology, providing a new lens through which we could understand cellular function, neural communication, and the functioning of various body systems such as the heart, fundamentally reshaping our understanding of life and biology.
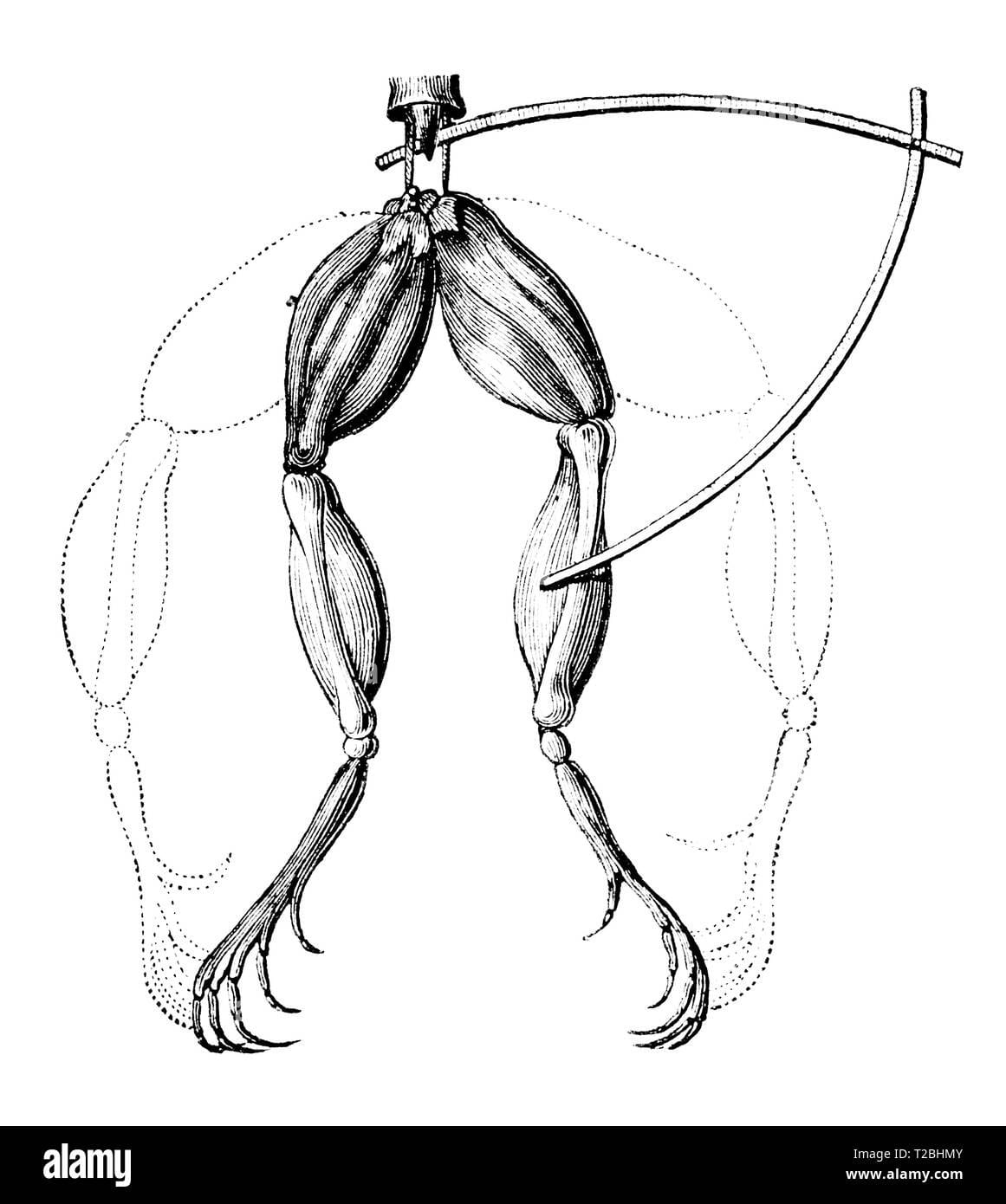
Galvani Frog experiment
Alessandro Volta, Galvani’s contemporary, disagreed with Galvani’s idea of “animal electricity” and proposed that the contractions Galvani saw were due to the metals he used to connect his apparatus, rather than the tissues themselves. This argument laid the groundwork for modern bioelectricity understanding, combining both theories.
In the 19th and 20th centuries, scientists discovered a phenomenon known as resting potential and action potential. Resting potential is the voltage across the cell membrane whilst inactive. The action potential is the rapid rise and subsequent fall of voltage during a state of activity. This creates an electric impulse that can transmit signals along nerves, which was modelled by Alan Hodgkin and Andrew Huxley in the Hodgkin-Huxley model of Action potentials.
Key Terms:
Bioelectricity: any electrical phenomenon that is actively generated by cells or that is applied to cells to affect cell phenotype. It typically involves movement of ions through ion channels.
Morphogenesis: the biological process that causes a tissue or organ to develop its shape by controlling the spatial distribution of cells during embryonic development.
Ion Channels: Protein structures that facilitate selective passage of ions across the cell membrane, modulating neuronal excitability, and are categorized into voltage-gated (responds to electrical potential within cell membrane) and ligand-gated (responds to specific chemical signals) based on opening mechanisms.
Resting Potential: The voltage across the cell membrane whilst inactive.
Action potential: The rapid rise and subsequent fall of voltage during a state of activity.
Intelligence: I am defining intelligence under the point of: can respond to problems without having been coded for by the gene. This means that anything which can react, acclimate, and alter itself rapidly is being called intelligent.
Micheal Levin’s research has 3 distinct points:
- Understanding how bodily cells form bioelectric networks for storing and recalling pattern memories that guide morphogenesis.
- Creating next generation AI tools for helping scientists understand top-down control of pattern regulation (a new bioinformatics of shape).
- Bioinformatics is a study merging computer science and biology, where bioinformaticians develop software for understanding biological data.
- Using these insights to enable new capabilities in regenerative medicine and engineering.
Bioelectricity in Nature
Bioelectricity plays a crucial role in most observable natural phenomena. Whether it is the shock of an electric eel, the healing of a wound, or a twitch of an eye, it is all controlled by bioelectricity. These phenomena expand to cell proliferation, differentiation, migration, and tissue patterning.
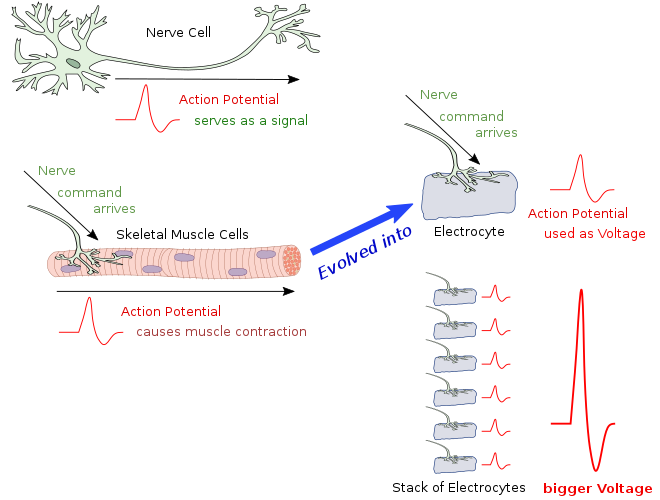
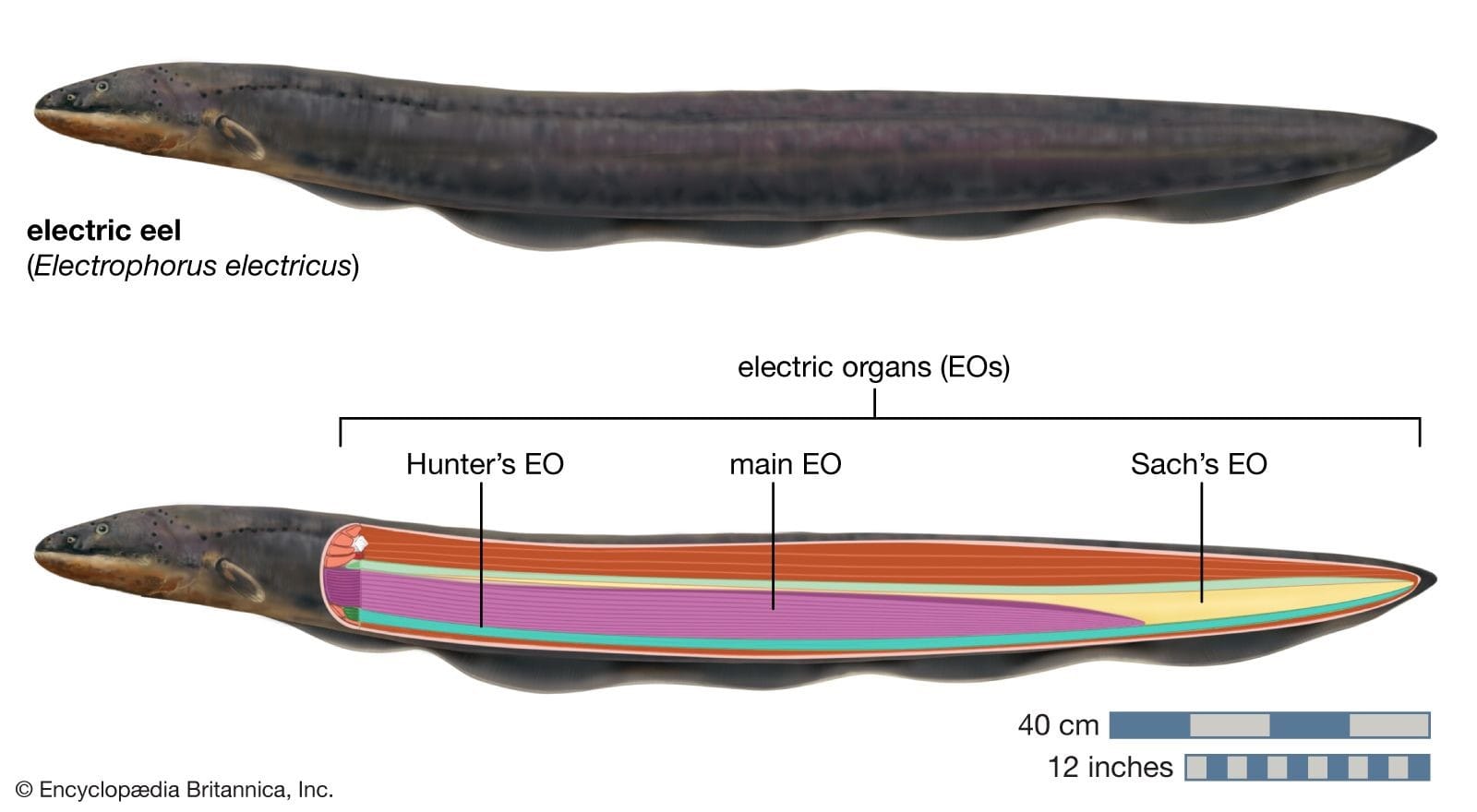
Here are some examples in nature:
- Electric Eels generate strong electric fields to locate prey and stun them. They have specialized cells called electrocytes, found in the dermis. They are stacked end to end and can produce a shock of up to 600 volts.
- Neurons communicate using bioelectricity, where they send impulses to each other by action potentials, which travel along the neurons' axons. At the synapses, it triggers the release neurotransmitters, which diffuse across to the receiver, where it is then creates the signal to send another impulse of bioelectricity.
- The heart relies on bioelectricity for each beat. A specialized group of cells, known as the sinoatrial node, generates an electrical impulse that spreads through heart muscle cells, causing them to contract and pump blood.
- When the skin is cut, the voltage across the epithelium is disrupted, creating an imbalance of ions. This behaves as a call to attention for other the "repair crew", which is drawn in by the voltage. As the wound gets resealed, the membrane potential dissipates and the bio-electric network can resume its work.
Michael Levin’s Research
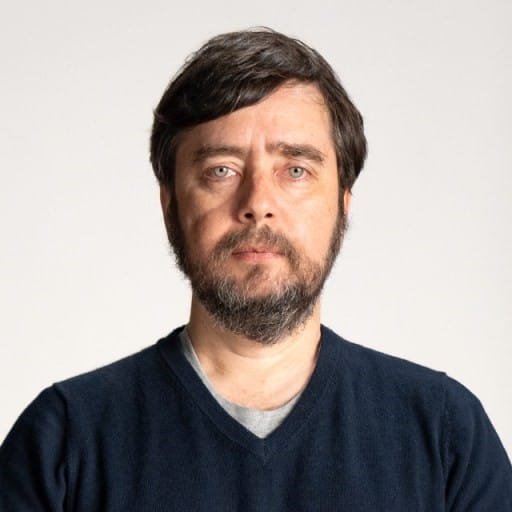
Michael Levin’s team at Tufts University explores bioelectricity, a groundbreaking approach in developmental biology and regenerative medicine. They study how cells and tissues use bioelectrical signals, not just chemical ones, to communicate and make decisions, which is crucial for development, regeneration, and repairing defects and injuries. Their experiments have shown the ability to manipulate these signals, leading cells to form new structures. This suggests an ‘innate intelligence’ within cells and tissues, a complex network of communication and decision-making at the cellular level driven by bioelectric signaling. This could revolutionize regenerative medicine, cancer biology, and synthetic bioengineering by providing a new understanding of cellular decision-making, potentially transforming our comprehension of life at the cellular level and the future of medicine.
Morphogenesis
There are a couple key aspects to account for. They are:
- Cell Differentiation, which is where cells become specialized, allowing them to develop into special tissues and organs within organisms.
- Cell Growth, cells divide and grow to increase the size of the organism.
- Cell death, programmed cell death (apoptosis) removes unnecessary cells and shapes structures.
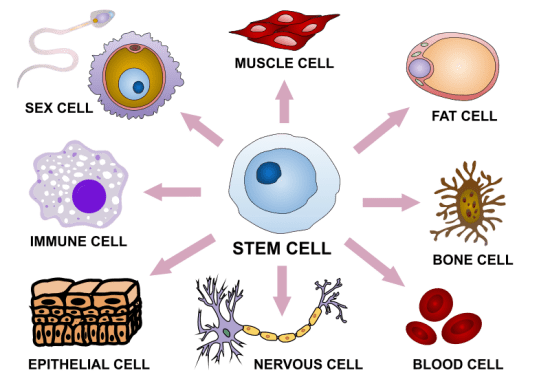
Different types of cell differentiation
Morphogenesis is essential for creating the complex, multicellular organisms we see in nature. It orchestrates the movement from a simple, undifferentiated mass of cells to a complex structure with specialized cells and tissues.
Levin’s research shows that bioelectric signals play a crucial role in morphogenesis. These signals guide cells to differentiate, creating the desired shapes and structures. This in-depth, bioelectrical understanding of morphogenesis could lead to huge breakthroughs in regenerative medicine and synthetic bioengineering.
Groundbreaking Experiments
Extra Eye on a Frog:
- In this experiment, Levin and his team were able to induce the growth of an extra eye on a frog embryo by manipulating bioelectric signals. They did this without altering any additional genes or materials. By changing the bioelectric code, they directed cells at a specific site to transform into eye cells. This experiment underscored the concept of "morphogenetic field" - an idea that cell groups can be influenced to change their behavior based on bioelectric signaling.
Tadpole's Eyes Shifted Back to Normal Position:
- In another study, Levin's team surgically repositioned the eyes of tadpoles to the tail region. They then used drugs to manipulate bioelectric signaling. The eyes migrated back to their correct anatomical position in the head over time. This demonstrated that bioelectric cues guide cells and tissues to their correct positions, an integral aspect of large-scale anatomical repair and body remodeling.
- The other version of this was a tadpole with a mutation that caused its facial features to be misaligned. The leading hypothesis till this point was that the features had a pre-coded movement plan, and that the face of this tadpole would still be wrong when it grew into a frog. They were proven wrong, however, when the face was normal as a frog. This challenged the previous hypothesis and showed that the cells had an innate “intelligence” about them and were capable of large-scale change without external prompting.
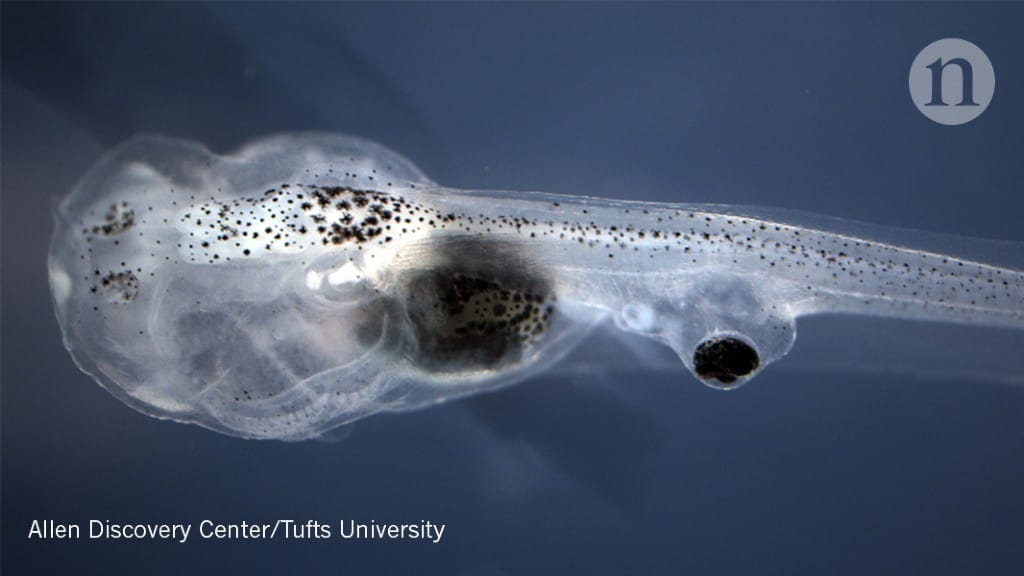
Planarians Regeneration:
- Planarians, a type of flatworm, are known for their impressive regenerative abilities. In a series of experiments, Levin manipulated their bioelectric signaling during the regeneration process. By doing this, he was able to make the planarians grow heads where tails should be, or grow additional heads, all without altering the worms' genome. This highlighted the importance of bioelectricity in regeneration and patterning and demonstrated that genomic information alone does not dictate the final structure of an organism.
All these experiments provide a clear proof-of-concept that cells and tissues use bioelectric cues to communicate and coordinate their activities, which has significant implications for developmental biology, regenerative medicine, and synthetic bioengineering.
Technical Aspects
- Understanding Bioelectric Patterning: At the cellular level, bioelectric signals help in guiding growth and development. This "bioelectric patterning" provides instructions to cells on what type of cell to become and where in the organism to form specific structures.
- Tools & Techniques: While exact tools aren't specified, Levin's work likely employs a combination of electrophysiological tools for measuring bioelectric signals, microscopic imaging for observation, and engineering techniques for modifying cells' bioelectric properties.
- Model Organisms: A range of organisms - from bacteria, slime molds, algae, to frogs, human cultured cells, and planaria - are used in this research to understand the universality and unique aspects of bioelectric communication in different species.
- Practical Experimentation: Levin's team has manipulated bioelectric patterning in planaria, resulting in two-headed worms from a single treatment. This shows the potential for altering developmental outcomes by modifying bioelectric signals.
Future Implications
- Michael Levin's research into bioelectric patterning holds substantial potential for transformative applications, particularly in the field of regenerative medicine and bioengineering. Some possible future implications could include:
- Regenerative Medicine: The ability to manipulate bioelectric signals to control cell growth and development could lead to breakthroughs in the field of regenerative medicine. We may be able to regenerate damaged or lost tissues, and even entire body parts, which could revolutionize treatments for a variety of injuries and illnesses.
- Cancer Treatment: Bioelectric patterns are disrupted in cancerous cells. Understanding these bioelectric disruptions could lead to new diagnostic techniques or therapies that restore healthy bioelectric patterns to cancerous tissues.
- Developmental Disorders: Understanding how bioelectric signals guide development could also shed light on developmental disorders. This could lead to potential treatments for conditions that are currently considered irreversible.
- Biological Robots (Xenobots): A deeper understanding of bioelectric signals could pave the way for the design and creation of novel, living machines, or "biological robots". This technology could be used in a variety of applications, from cleaning up environmental waste to delivering drugs within the body.
- Basic Understanding of Life: On a more fundamental level, Levin's research challenges our understanding of life and the boundaries between different forms of life. It opens up questions about consciousness, intelligence, and the nature of life itself, with implications that could reverberate across many fields of study.
Of course, this research is still in the early stages, and these potential applications will require substantial further study to become reality. However, the work of Levin and his team is undoubtedly a significant step toward a future where we have much greater control over biological development and regeneration.
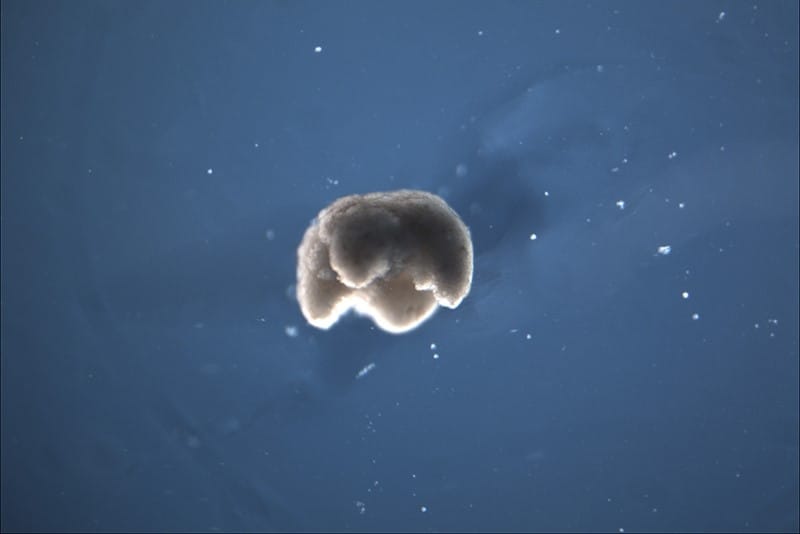